Quantum Gyroelectric Effect: How Photon Spin Could Revolutionize Future Tech
"New research explores photon spin-1 quantization in topological bosonic phases, paving the way for groundbreaking advancements in material science and technology."
For decades, scientists studying matter have been fascinated by "topological phases". These phases are unique states of matter with properties protected by their fundamental structure. Imagine a donut; its topology remains the same whether you stretch or bend it, as long as you don't create or close any holes. Topological phases of matter exhibit similar robustness, making them incredibly promising for developing new technologies.
Traditionally, research in this field has focused on "fermions," particles like electrons that have half-integer spins. Spin, in quantum mechanics, is a fundamental property of particles that dictates how they interact with magnetic fields. But what about "bosons," particles like photons that have integer spins? Scientists have long wondered if bosonic systems could also exhibit topological phases and what unique properties these phases might possess.
Now, a groundbreaking study from Purdue University delves into the world of topological bosonic phases, revealing the secrets of "photon spin-1 quantization." This research not only deepens our understanding of light-matter interactions but also opens exciting new avenues for technological innovation. This innovative new method could be the key to unlocking the next generation of advanced tech.
Unlocking the Secrets of Photon Spin: What is Spin-1 Quantization?
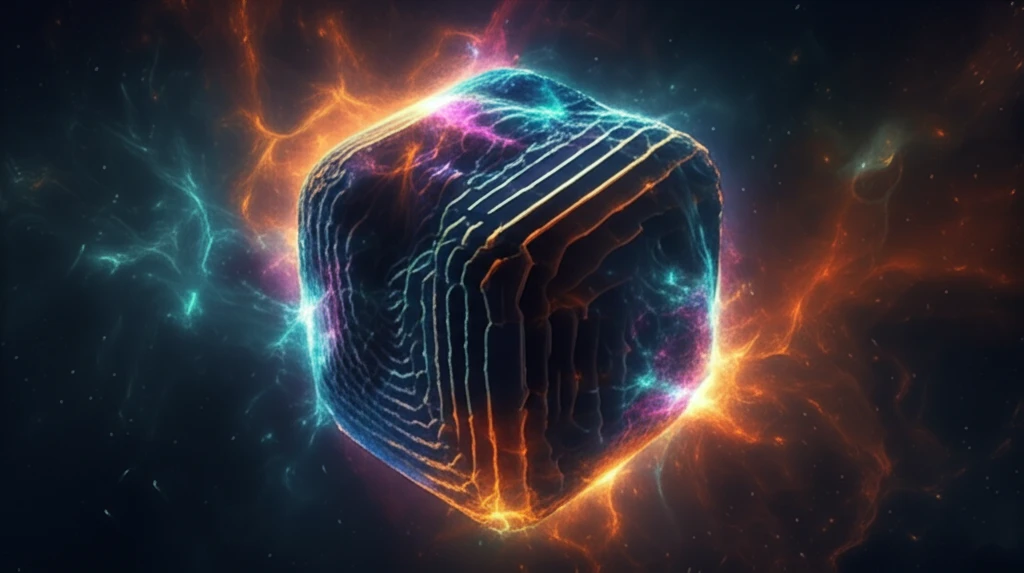
In the quantum world, spin isn't just about things physically spinning. It's an intrinsic form of angular momentum that dictates how a particle interacts with its environment. Fermions, like electrons, have a spin of 1/2, meaning they have to be rotated twice (720 degrees) to return to their original state. Bosons, on the other hand, have integer spins. Photons, the particles that make up light, have a spin of 1.
- Transverse Spin Quantization: The study demonstrated that in topological bosonic phases, photons exhibit "transverse spin-1 quantization." This means the spin of the photon is aligned perpendicular to its direction of motion and can only take on specific, quantized values.
- Unique Bosonic Phases: The researchers predicted two unique bosonic phases based on this spin quantization: one with even parity (C = ±2) and one with odd parity (C = ±1). Parity refers to how a system's properties change when its spatial coordinates are inverted.
- Edge States: A key finding was the existence of a single, gapless photon at the edge of the even parity phase (C = ±2). This unidirectional photon is "spin-1 helically quantized," meaning its spin is locked to its direction of motion, making it immune to backscattering and defects.
What Does This Mean for the Future?
This research provides a theoretical framework for understanding and exploring topological bosonic phases, paving the way for future discoveries and technological innovations. The ability to control and manipulate light at this fundamental level could revolutionize various fields, including: <ul> <li><b>Advanced Materials:</b> Designing new materials with unprecedented optical properties.</li> <li><b>Quantum Computing:</b> Developing robust quantum systems that are less susceptible to noise.</li> <li><b>High-Speed Communication:</b> Creating faster and more efficient communication technologies.</li> </ul> While this research is still in its early stages, it represents a significant step forward in our understanding of light-matter interactions and has the potential to transform technology as we know it. This will involve momentum-resolved electron energy-loss spectroscopy and cold atom near-field measurement of nonlocal optical Hall conductivity.