Unlocking the Secrets of Chemical Reactions: How Vibrational Energy Changes the Game
"Dive into the groundbreaking study exploring how vibrational energy impacts the C+OH reaction, altering product formation and shedding light on molecular dynamics."
In the realm of chemistry, understanding how reactions occur at the molecular level is crucial. Chemical reactions, the fundamental processes that drive everything from the synthesis of new materials to the energy production in our bodies, are governed by a complex interplay of factors. Among these, the energy states of the reacting molecules play a pivotal role. Recent research has shed light on the significant impact of vibrational energy—the energy associated with the internal vibrations of molecules—on the dynamics and outcomes of a specific reaction: C + OH → CO + H.
The reaction between a carbon atom (C) and a hydroxyl radical (OH) is a fundamental process with implications in various fields, including combustion chemistry and atmospheric science. This seemingly simple reaction can follow different pathways, leading to different energy distributions in the products (carbon monoxide, CO, and a hydrogen atom, H). What makes this reaction particularly intriguing is the influence of vibrational energy in the OH molecule. By exciting the OH molecule to higher vibrational states, scientists can alter the course of the reaction, favoring certain products and energy distributions over others.
A new study published in The European Physical Journal D delves deep into the intricacies of this reaction, using advanced computational methods to explore how vibrational energy affects the reaction dynamics and the final energy states of the products. This article translates the complex findings of this research into an accessible format, highlighting the key insights and their potential implications.
The Experiment: Probing the C + OH Reaction
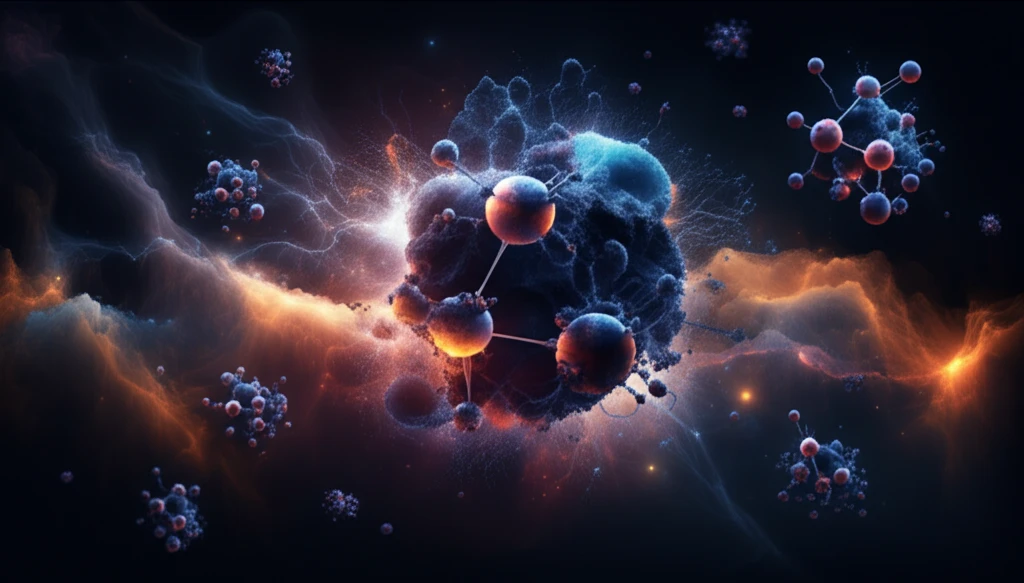
The research team focused on the reaction between carbon atoms (C) and hydroxyl radicals (OH), specifically examining how pre-existing vibrational energy within the OH molecule influences the reaction pathway. They used sophisticated quantum mechanical calculations to simulate the reaction, tracking the energy as the reaction unfolds.
Implications and Future Directions
This study provides valuable insights into the role of vibrational energy in chemical reactions. By demonstrating how vibrational excitation can alter reaction pathways and product energy distributions, it underscores the importance of considering molecular energy states in chemical modeling and reaction control. This knowledge could be applied to optimize combustion processes, design more efficient chemical synthesis routes, and better understand atmospheric chemistry. Future research could explore the effects of other forms of energy, such as rotational energy, and investigate more complex reaction systems to further refine our understanding of molecular dynamics.