Unlocking Nuclear Secrets: How a Tiny Atom Could Rewrite Physics Textbooks
"Scientists are testing the limits of the Brink-Axel hypothesis, revealing new insights into the behavior of atomic nuclei and its impact on our understanding of nuclear physics."
For decades, scientists have leaned on certain assumptions about how atomic nuclei behave, especially when they're in an excited state. One of the most vital of these assumptions is the idea that the way a nucleus releases energy—specifically through gamma decay—doesn't depend on how that nucleus was initially energized. This concept, known as the generalized Brink-Axel (gBA) hypothesis, simplifies a lot of complex calculations, making it easier to predict nuclear reactions. However, like any good scientific theory, it needs constant testing.
Imagine the nucleus of an atom as a black box. You pump energy into it, and it spits that energy out in the form of gamma rays. The gBA hypothesis says that the characteristics of those gamma rays depend only on their energy and not on the specific way you excited the nucleus in the first place. This is a handy rule, but what if it's not always true? What if the internal structure of the nucleus and its energy state do influence the gamma decay process? That's what a team of physicists recently set out to investigate.
In a study published in Physical Review C, researchers meticulously examined the gamma decay patterns of nickel isotopes, specifically 64Ni and 65Ni. By smashing particles into these isotopes and then carefully measuring the emitted gamma rays, they sought to determine whether the gBA hypothesis holds up under close scrutiny. Their findings could have significant implications for fields ranging from nuclear astrophysics to reactor physics, potentially reshaping how we model nuclear behavior in extreme conditions.
The Experiment: Probing Nickel with Particles
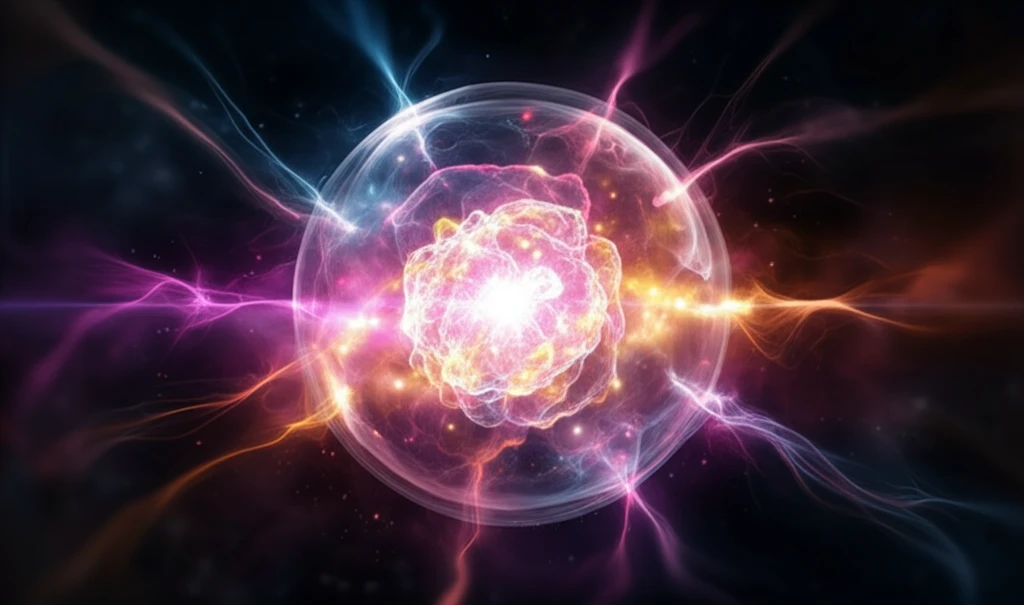
The team's approach involved analyzing existing data from experiments conducted at the University of Oslo. These experiments used particle-gamma coincidence techniques. Think of it this way: they shot a beam of particles at a target of nickel isotopes and measured not only the outgoing particles but also the gamma rays emitted in coincidence. This allowed them to correlate the energy of the incoming particles with the energy and characteristics of the emitted gamma rays, providing a detailed picture of the nuclear decay process.
- The relationship between initial excitation energy and gamma-ray strength.
- How well the data aligned with the generalized Brink-Axel hypothesis.
- Fluctuations in transition strengths as a function of gamma-ray and excitation energy.
What It All Means: A Step Forward, But More to Explore
The research supports the idea that the gamma strength functions of 64Ni and 65Ni are largely independent of the initial excitation energy, aligning with the generalized Brink-Axel hypothesis. However, they also observed significant fluctuations, particularly when looking at decays to the ground state and first excited states. These fluctuations suggest that the nuclear structure and the specific energy levels involved can indeed influence the gamma decay process, especially at lower excitation energies. This nuanced understanding is crucial for refining nuclear models and improving predictions in various applications.