Unlocking Fusion Energy: How Plasma Research is Forging a Sustainable Future
"Delve into the groundbreaking studies using high-flux plasma to understand and enhance the durability of fusion reactor materials, paving the way for cleaner energy solutions."
In an era defined by the urgent need for sustainable energy solutions, fusion energy stands out as a promising contender. Harnessing the power that fuels the stars, fusion offers the potential for virtually limitless, clean energy. However, realizing this potential requires overcoming significant engineering challenges, particularly in the materials science domain. The heart of a fusion reactor, where atoms are forced together at extreme temperatures to release energy, demands materials that can withstand unprecedented conditions.
Tungsten, known for its exceptional heat resistance and high melting point, has emerged as a primary candidate for the divertor material in fusion reactors like ITER (International Thermonuclear Experimental Reactor) and future DEMO reactors. The divertor is a critical component that handles the intense heat and particle flux generated during fusion reactions. Understanding how tungsten behaves under these extreme conditions is paramount to ensuring the longevity and efficiency of fusion reactors.
Recent research focusing on the effects of high-flux plasma on tungsten microstructure sheds light on the material's response to fusion-relevant conditions. By employing transmission electron microscopy (TEM), scientists are gaining unprecedented insights into the changes occurring at the atomic level, paving the way for optimizing material performance and enhancing the feasibility of fusion energy.
High-Flux Plasma: A Microscopic Examination
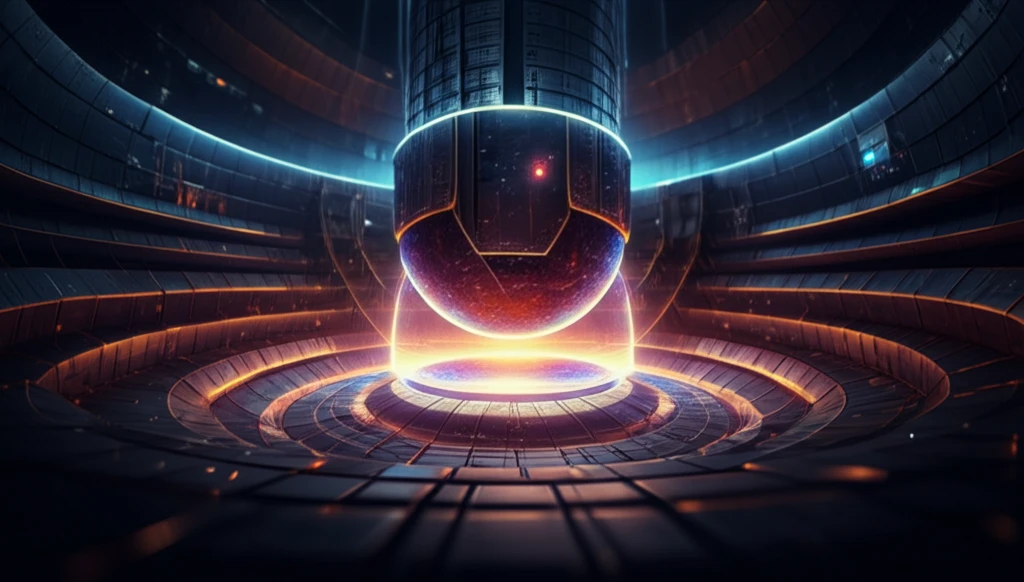
The study meticulously examines the microstructural changes in tungsten samples subjected to high-flux plasma, simulating the harsh conditions within a fusion reactor. Researchers analyzed both annealed and heavily deformed tungsten states to understand how initial material conditions influence its response to plasma exposure. The experiments were conducted using the Pilot-PSI accelerator, which generates a low-energy, high-density deuterium plasma.
- Significant increase in dislocation density in surface layers.
- Plastic deformation induced by thermal shocks and deuterium penetration.
- Formation of dislocation loops and aggregates near grain boundaries.
- Microstructural changes diminish at depths greater than 10-15 µm from the surface.
Toward a Sustainable Fusion Future
The insights gained from this research are crucial for the advancement of fusion energy technology. By understanding how high-flux plasma affects the microstructure of tungsten, scientists and engineers can develop strategies to enhance the durability and performance of materials used in fusion reactors. This knowledge contributes to the feasibility of fusion as a clean, sustainable energy source for the future, offering a pathway to meet the growing global energy demands while minimizing environmental impact. As research continues and technology evolves, fusion energy may soon become a cornerstone of a sustainable and secure energy future.