Unlock the Power of Visible Light: How New Materials are Revolutionizing Clean Energy
"Scientists are optimizing the synthesis of zinc-rich gallium zinc oxynitrides, paving the way for more efficient photocatalytic water splitting and sustainable hydrogen production."
Imagine a world powered by clean, renewable energy, where hydrogen fuel is as common as gasoline is today. This vision hinges on our ability to efficiently harness solar energy to split water into hydrogen and oxygen—a process known as photocatalytic water splitting (OWS). While many materials can perform this trick, most require ultraviolet (UV) light, which makes up only a small fraction of the solar spectrum. The quest for materials that can tap into the more abundant visible light is driving innovation in sustainable energy.
Oxynitrides, compounds that combine oxygen and nitrogen, have emerged as promising candidates. These materials boast a narrow bandgap, allowing them to absorb visible light, and they are stable under irradiation, overcoming the limitations of other potential solutions. Among these, gallium zinc oxynitride (GaN:ZnO) stands out as a photocatalyst capable of stoichiometric OWS when loaded with a catalyst like ruthenium oxide (RuO2).
However, synthesizing GaN:ZnO with high efficiency has been a challenge. Traditional high-temperature methods often lead to significant zinc loss due to its volatility at elevated temperatures, limiting the material's performance. Researchers are now exploring novel synthesis routes to overcome this hurdle and unlock the full potential of GaN:ZnO.
Optimizing Synthesis for Enhanced Performance
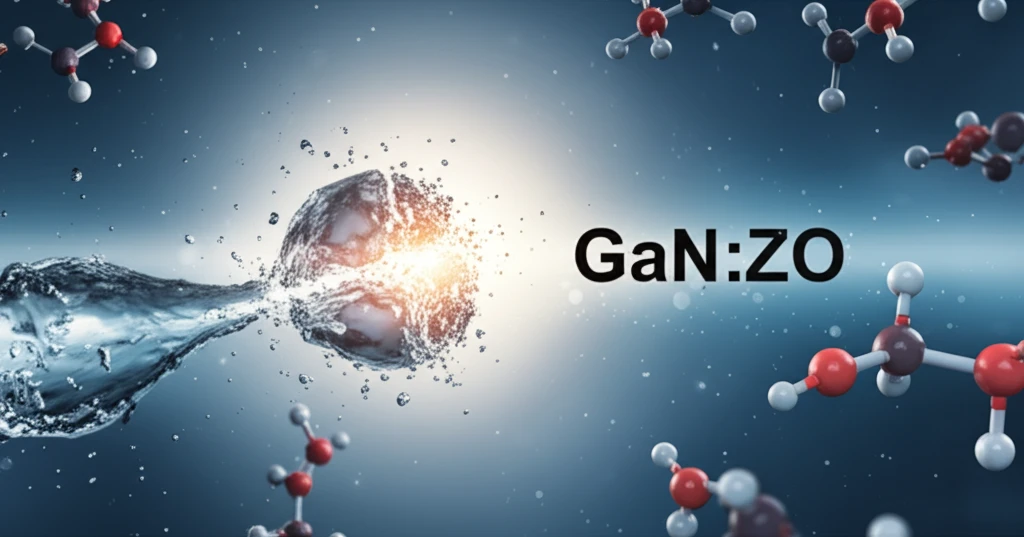
A recent study published in ZAAC: Journal of Inorganic and General Chemistry details a method for optimizing the synthesis of zinc-rich GaN:ZnO by combining co-precipitation and moisture-assisted nitridation. The conventional high-temperature solid-state synthesis (HTS) approach, first employed by Domen and co-workers, involves heating a mixture of β-Ga2O3 and ZnO powder in a high-ammonia stream at temperatures up to 850°C. This method, however, results in substantial zinc loss, limiting the achievable zinc content in the final material.
- Co-precipitation: Ensures even distribution of Ga and Zn.
- LDH Structures: Facilitate ammonia diffusion during nitridation.
- Moisture-Assisted Nitridation: Reduces zinc loss and enhances material properties.
- Lower Temperatures: Reduces energy consumption and material degradation.
Future Implications and Sustainability
The optimized synthesis method paves the way for creating more efficient and cost-effective GaN:ZnO photocatalysts. By minimizing zinc loss and maximizing zinc content, researchers can fine-tune the material's bandgap, enhancing its ability to absorb visible light. This breakthrough brings us closer to realizing the potential of photocatalytic water splitting as a sustainable source of hydrogen fuel, contributing to a cleaner, more secure energy future. Further research will focus on correlating the structural properties of GaN:ZnO samples with their photocatalytic activity, aiming to refine the synthesis process and unlock even greater efficiencies.