Unlock Quantum Potential: How Silicon Carbide Defects Could Revolutionize Computing
"Scientists are exploring the unique properties of divacancy defects in silicon carbide to pave the way for more powerful quantum computers and advanced sensing technologies. Learn how this research brings quantum computing closer to reality."
The quest for creating efficient and stable qubits—the fundamental building blocks of quantum computers—is a central challenge in modern science. Unlike classical bits that store information as 0s or 1s, qubits can exist in a superposition of both states, allowing quantum computers to perform complex calculations far beyond the reach of today's machines. This capability promises breakthroughs in fields ranging from medicine and materials science to artificial intelligence and cryptography.
Researchers are actively exploring diverse materials and methods to create reliable qubits. Among the most promising candidates are point defects within semiconductors and insulators. These defects, which include vacancies, antisites, and substitutional impurities, can behave like isolated atoms with well-defined quantum states. This makes them suitable for quantum computing applications, as they can be controlled and manipulated using various techniques.
One such material gaining significant attention is silicon carbide (SiC). Known for its wide bandgap and ability to be grown in large single crystals, SiC hosts a variety of stable defects that are being considered as potential qubits. This article delves into recent research focused on the divacancy defect in 4H-SiC, exploring how manipulating its charge states could lead to advancements in quantum technology.
The Promise of Divacancies in 4H-SiC
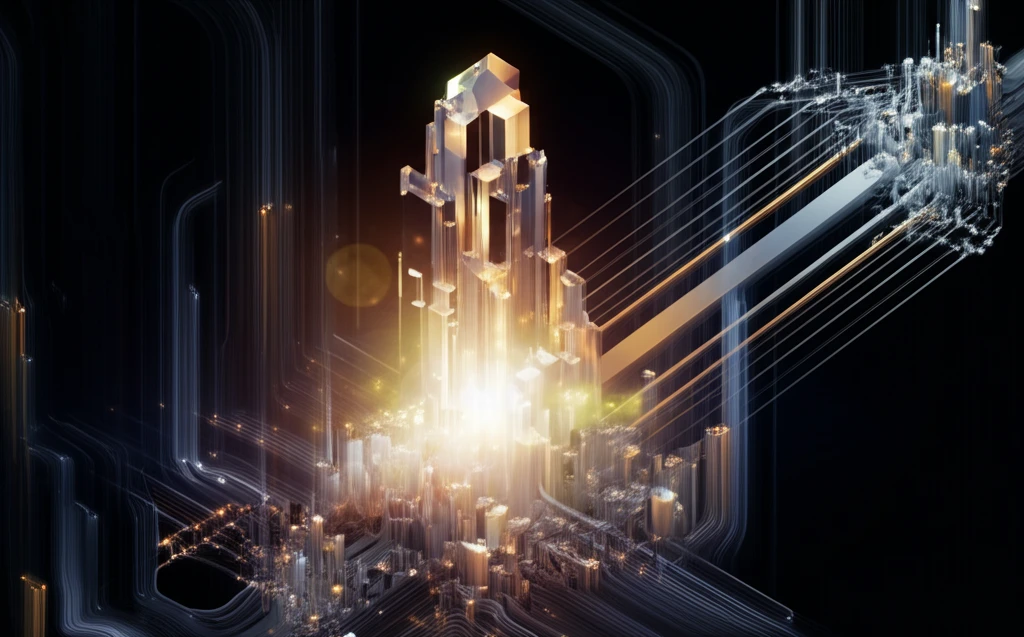
The neutral divacancy in 4H-SiC has emerged as a particularly promising candidate for quantum computing. Its localized triplet ground state can be optically spin-polarized, similar to the well-known nitrogen-vacancy (NV) center in diamond. After initialization, the qubit states can be coherently manipulated using pulsed microwaves, and their spin states can be read out by measuring the photoluminescence intensity after electronic excitation.
- Localized triplet ground state allows optical spin polarization.
- Coherent manipulation via pulsed microwaves.
- Spin states can be read out through photoluminescence intensity.
- Multiple charge states impact qubit performance.
Unlocking the Future of Quantum
Continued research into the charge state dynamics of divacancies in silicon carbide holds immense promise for advancing quantum computing. By gaining a deeper understanding of the underlying mechanisms and developing precise control over these defects, scientists are paving the way for more robust and scalable quantum technologies. This journey into the quantum realm promises to unlock unprecedented computational power and revolutionize industries across the board.