Unlock Quantum Potential: How Cold Development Revolutionizes Chip Manufacturing
"A deep dive into the innovative cold-development technique, enhancing the fabrication of Josephson junctions for advanced quantum computing and superconducting circuits."
Quantum computing, once a theoretical dream, is rapidly becoming a tangible reality, fueled by advancements in superconducting circuits. At the heart of these circuits lie Josephson junctions, tiny devices that act as the quantum equivalent of switches. Their performance critically impacts the stability and coherence of quantum bits (qubits), the fundamental building blocks of quantum computers. These junctions are frequently constructed using aluminum and aluminum oxide (Al/AlOx/Al) due to their relatively long coherence times and ease of manufacture.
Fabricating these junctions, however, is no small feat. The conventional methods often struggle with precision and reliability at the nanoscale, hindering the overall progress of quantum computing. Factors like the quality of substrate materials, such as silicon and sapphire, and the intricacies of fabrication processes play critical roles. The goal is to create junctions with well-defined geometries and minimal defects to maximize qubit coherence – the time during which a qubit can reliably perform calculations.
Now, a new approach is gaining traction: cold development. This technique, involving extremely low temperatures during the fabrication process, promises to significantly enhance the precision and repeatability of creating Josephson junctions, paving the way for more robust and powerful quantum computers.
The Chilling Effect: How Cold Development Works
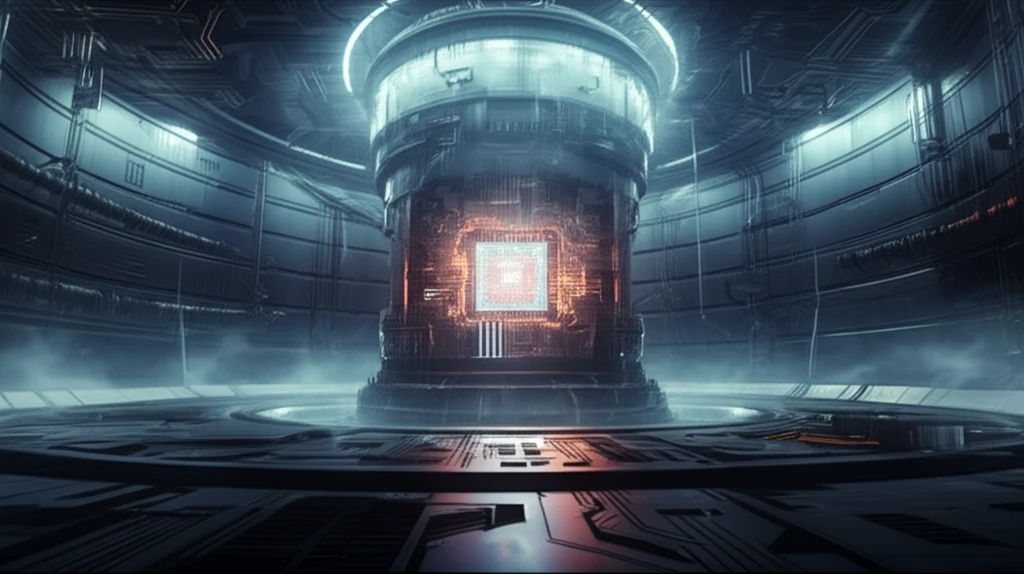
Cold development is a fabrication process carried out at temperatures as low as -20°C. The primary goal is to improve the electron-beam lithography (EBL) process, which is used to create the incredibly small patterns needed for Josephson junctions. During EBL, a beam of electrons etches a pattern onto a resist layer, typically made of materials like methyl methacrylate (MMA) and polymethyl methacrylate (PMMA).
- Reduced Sensitivity: The resist becomes less reactive to the developer, providing finer control over the etching process.
- Minimized Imperfections: Fewer molecules are washed away from the edges of the etched trenches, resulting in sharper and more defined patterns.
- Enhanced Repeatability: The process becomes more consistent, ensuring that each junction is nearly identical to the last.
- Increased Dose Margins: The range of acceptable electron beam exposure doses widens, making the process more forgiving and easier to optimize.
A Quantum Leap Forward
The development and application of cold development techniques mark a significant step towards creating more reliable and efficient quantum computers. By improving the fabrication of Josephson junctions, this method addresses a critical bottleneck in quantum technology. As research continues and these techniques are refined, we can expect to see even more powerful and stable quantum systems emerge, unlocking unprecedented computational capabilities and pushing the boundaries of scientific discovery.