Unlock Battery Potential: How Anion Alloying Could Revolutionize Solid-State Batteries
"Discover how anion alloying in lithium indium halides can enhance superionic conductivity, paving the way for safer, more efficient all-solid-state batteries."
The quest for better batteries is a constant one, driven by our ever-increasing reliance on portable electronics, electric vehicles, and renewable energy storage. While lithium-ion batteries have become ubiquitous, they still have limitations in terms of safety, energy density, and lifespan. This has fueled intensive research into alternative battery technologies, with all-solid-state batteries emerging as a particularly promising candidate.
All-solid-state batteries offer several advantages over their liquid electrolyte counterparts. By replacing the flammable liquid electrolyte with a solid material, the risk of fires and explosions is significantly reduced. Furthermore, solid electrolytes can potentially enable the use of higher-energy electrode materials, leading to batteries with greater energy density and improved performance. One of the key challenges in developing all-solid-state batteries is finding solid electrolytes with sufficiently high ionic conductivity—that is, the ability for lithium ions to move easily through the material.
Now, a new approach has emerged as a promising strategy for tuning the ionic conductivity of solid electrolytes: anion alloying. By combining different anions (negatively charged ions) within the electrolyte material, researchers can manipulate its structural and chemical properties to enhance ion transport. A recent study published in APL Materials explores the effects of anion alloying in lithium indium halides, shedding light on the underlying mechanisms that govern ionic conductivity in these materials.
The Science of Anion Alloying in Lithium Indium Halides
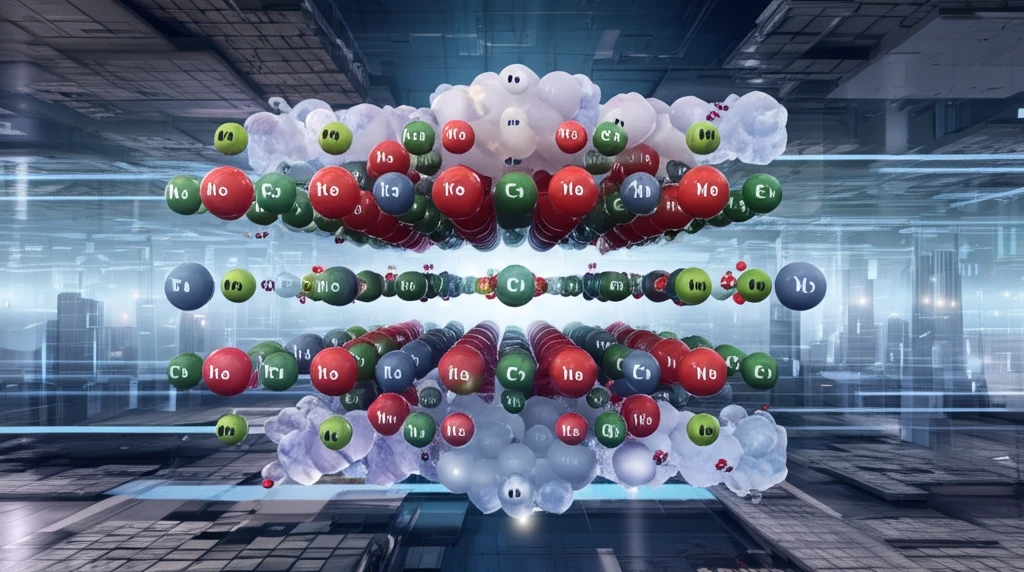
The research focuses on lithium indium halides (Li3InBr6-xClx), where bromine (Br) and chlorine (Cl) are combined in different proportions. The scientists employed a combination of first-principles molecular dynamics simulations and electronic structure analysis to understand how the alloying of anions affects the material's properties. These advanced computational techniques allow researchers to simulate the behavior of atoms and ions within the material, providing insights into the factors that influence ionic conductivity.
- Strain: Alloying introduces strain into the crystal lattice, which can either enhance or hinder ion transport depending on the specific composition.
- Bond Chemistry: The nature of the chemical bonds between lithium ions and the anions plays a crucial role in determining the ease with which ions can move through the electrolyte.
- Microstructure: The arrangement of different regions within the material, such as Br-rich and Cl-rich domains, can significantly impact ionic conductivity.
The Future of Solid-State Batteries is Bright
This research provides valuable insights into the complex interplay of factors that govern ionic conductivity in anion-alloyed solid electrolytes. By understanding these mechanisms, scientists can design and develop new materials with enhanced performance for all-solid-state batteries. As the demand for safer, more efficient, and longer-lasting batteries continues to grow, the development of advanced solid electrolytes will be crucial in unlocking the full potential of this game-changing technology. Anion alloying offers a promising pathway toward achieving this goal, bringing us closer to a future powered by all-solid-state batteries.