Tokamak Tech: Will 3D Instabilities Power Tomorrow's Fusion?
"New research on Helically Localized Ballooning Instabilities (HLBI) explores how 3D tokamak pedestals could unlock fusion energy, and what it means for the future of clean power."
The quest for sustainable and clean energy sources has led scientists and engineers down numerous paths, each with its own set of challenges and potential rewards. Among these, nuclear fusion stands out as a particularly promising candidate, offering the potential for nearly limitless energy with minimal environmental impact. Tokamak reactors, a leading design in fusion energy research, are at the heart of this endeavor. However, achieving stable and efficient fusion reactions within these devices requires a deep understanding and control of plasma instabilities.
Recent research has shed light on a particularly interesting phenomenon known as Helically Localized Ballooning Instabilities (HLBI) occurring within three-dimensional tokamak pedestals. These instabilities, which arise in the edge of the plasma, can significantly affect the performance and stability of the fusion reaction. Understanding and controlling HLBI is crucial for optimizing tokamak reactor designs and moving closer to practical fusion energy.
This article delves into the groundbreaking research on HLBI, exploring the underlying physics, experimental observations, and potential implications for the future of fusion energy. By examining how these instabilities form and behave, we can gain valuable insights into the complex dynamics of tokamak plasmas and pave the way for more efficient and reliable fusion reactors.
What Are Helically Localized Ballooning Instabilities (HLBI)?
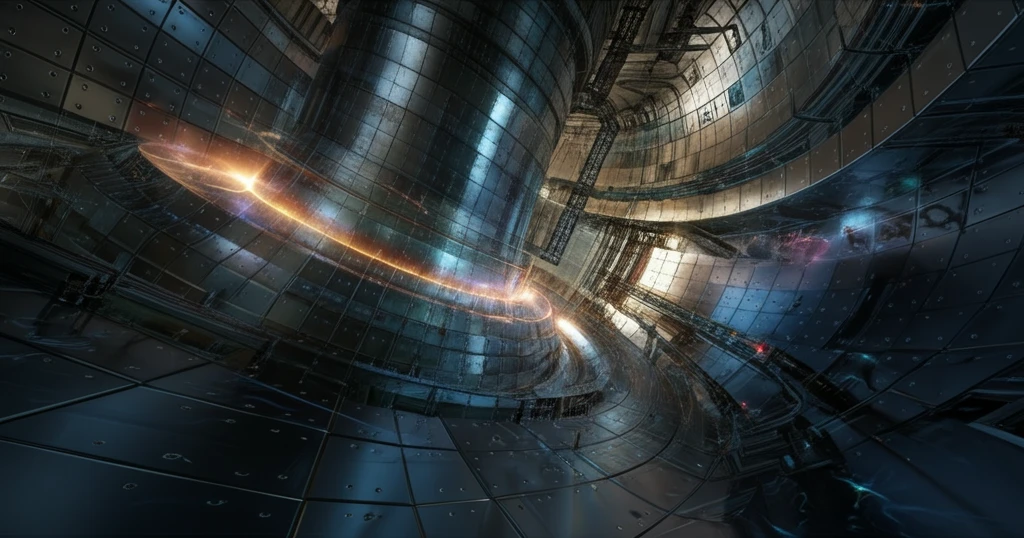
Helically Localized Ballooning Instabilities (HLBI) are a type of magnetohydrodynamic (MHD) instability that can occur in the plasma edge of tokamak reactors, particularly in configurations known as three-dimensional (3D) tokamak pedestals. To understand this, it's helpful to break down the key components:
- Magnetohydrodynamic (MHD): MHD studies the behavior of electrically conducting fluids (like plasma) in the presence of magnetic fields. MHD instabilities are disruptions in the plasma caused by imbalances in magnetic and pressure forces.
- Ballooning Instabilities: These instabilities are so named because they tend to 'balloon' outwards along magnetic field lines where the curvature is unfavorable for stability. They're driven by the pressure gradient in the plasma.
- Helically Localized: Unlike simpler, symmetrical instabilities, HLBI are localized in specific regions of the tokamak, often due to the complex 3D shaping of the magnetic fields. The 'helical' aspect refers to the spiral-like path that magnetic field lines trace around the tokamak.
- 3D Tokamak Pedestals: Modern tokamaks often employ non-axisymmetric magnetic fields to improve plasma confinement and stability. These 3D fields create variations in the plasma edge, influencing the behavior of instabilities.
HLBI: A Step Toward Fusion
Research into Helically Localized Ballooning Instabilities represents a critical step towards realizing the potential of fusion energy. By understanding the complex dynamics of plasma instabilities and developing methods to control them, scientists and engineers are paving the way for more efficient, stable, and reliable tokamak reactors. While challenges remain, the progress made in this field offers a beacon of hope for a future powered by clean, sustainable fusion energy.