Hidden Heat Traps: How Native Oxide Changes Everything You Thought You Knew About Silicon
"New research reveals the surprising impact of native oxide layers on thermal conductivity in silicon membranes, challenging conventional wisdom and opening doors to better thermal management in nano-devices."
Silicon is a foundational material in modern electronics, renowned for its semiconducting properties that enable the creation of transistors and integrated circuits. For decades, scientists and engineers have studied its properties extensively, leading to significant advancements in computing, communication, and countless other technologies. However, despite this deep understanding, surprises still emerge, particularly when examining silicon at the nanoscale.
One such surprise involves the "native oxide" layer that naturally forms on silicon surfaces when exposed to air. This layer, composed primarily of silicon dioxide (SiO2), has generally been considered an unavoidable nuisance or a stable protective coating. However, new research is revealing that this native oxide plays a far more significant role in silicon's thermal properties than previously thought, especially in nanoscale structures like silicon membranes.
Recent studies highlighted in Applied Physics Letters, are demonstrating that this oxide layer can dramatically alter how heat flows through these tiny structures, impacting their performance and reliability. This discovery challenges conventional wisdom and opens new avenues for optimizing thermal management in nano-devices, with implications for everything from faster processors to more efficient sensors.
The Unexpected Impact of Native Oxide
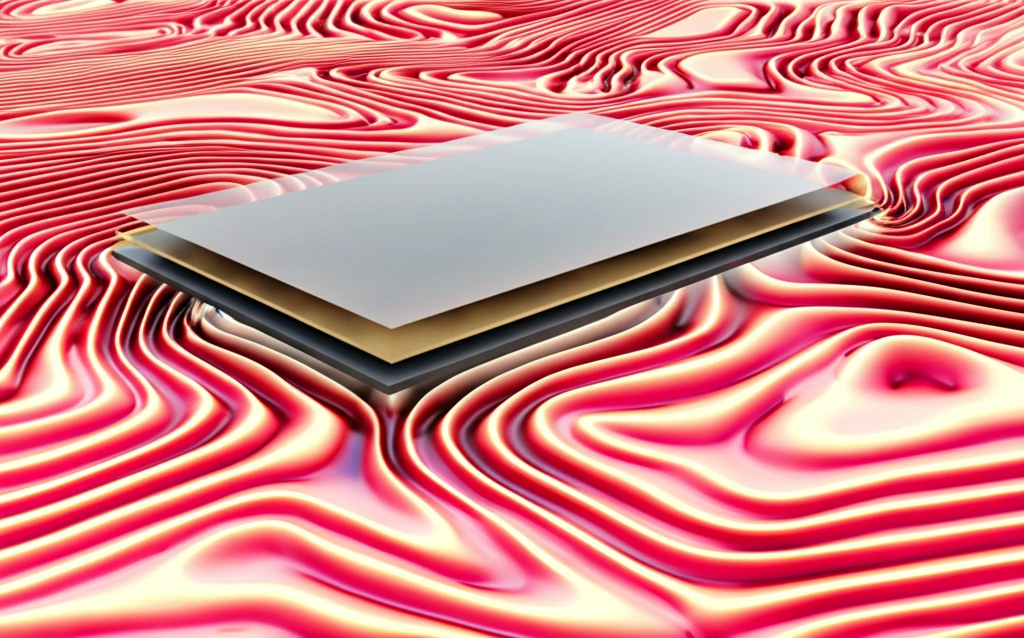
The research focuses on suspended silicon membranes, ultra-thin sheets of silicon that are isolated from a substrate, allowing for precise control and measurement of their properties. By using a technique called scanning thermal microscopy (SThM), scientists can map the temperature distribution across these membranes with high spatial resolution. This allows them to directly measure how effectively heat is conducted through the material.
- Interface Resistance: The boundary between the silicon and the silicon dioxide introduces a thermal resistance, hindering the movement of phonons (the primary carriers of heat in solids).
- Phonon Scattering: The disordered structure of the amorphous oxide layer causes phonons to scatter, reducing their mean free path and thus lowering thermal conductivity.
- Cross-Plane Transport: The oxide layer affects not only in-plane heat conduction but also cross-plane transport, meaning heat flow perpendicular to the membrane surface is also restricted.
- Thickness Matters: Even a very thin layer of native oxide (around 1.5 nm) can have a disproportionately large impact on thermal transport.
Implications and Future Directions
The discovery of the native oxide's significant impact on thermal transport in silicon membranes has profound implications for the design and optimization of nano-devices. As devices continue to shrink in size, the surface area to volume ratio increases, making surface effects like the presence of native oxide even more critical. Engineers must now consider the thermal properties of this oxide layer when designing nanoscale components to prevent overheating and ensure reliable performance. Future research will likely focus on developing methods to control or mitigate the effects of the native oxide. This could involve techniques such as surface passivation, where the oxide layer is modified to improve its thermal conductivity, or the use of alternative materials with more favorable thermal properties. Understanding and managing the thermal behavior of native oxide is crucial for unlocking the full potential of silicon in future technologies.