Cooling Quantum Gases: Unlocking Secrets of Superconductivity
"New research cools quantum gases to near absolute zero, offering unprecedented insights into exotic states of matter and high-temperature superconductivity."
In the realm of physics, the quest to understand the behavior of matter at its most fundamental level often leads scientists to explore extreme conditions. One such area of exploration involves cooling gases to temperatures near absolute zero, where quantum effects dominate. Recent research has achieved a significant breakthrough in this field, successfully cooling quantum gases to long-range antiferromagnetic order, a state where electron spins align in a repeating, opposite pattern.
This achievement marks a crucial step forward in the study of exotic states of matter and could pave the way for advancements in various technological applications, including quantum computing and materials science. The ability to control and manipulate matter at such low temperatures allows scientists to probe the intricate relationships between particles and gain insights into phenomena that are otherwise hidden at higher temperatures.
The driving force behind this research is the potential to unlock the secrets of high-temperature superconductivity. Superconductivity, the ability of a material to conduct electricity with no resistance, holds immense promise for energy-efficient technologies. However, the mechanisms behind high-temperature superconductivity remain largely mysterious. By studying quantum gases at extremely low temperatures, researchers hope to mimic the behavior of electrons in superconducting materials and gain a deeper understanding of the underlying physics.
The Significance of Antiferromagnetic Order
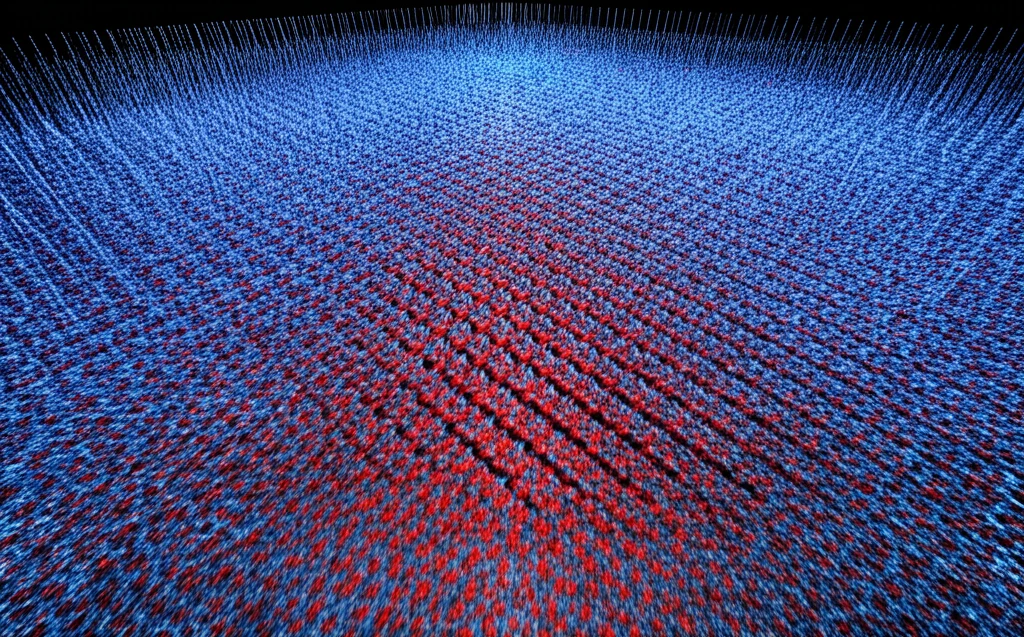
The observation of antiferromagnetic order in a lattice of ultracold atoms is a significant milestone. In this state, the spins of neighboring atoms align in opposite directions, creating a checkerboard pattern. This ordered arrangement arises from the interactions between the atoms, even though they are not directly touching. The ability to achieve and observe this state in a controlled environment provides a unique opportunity to study the fundamental principles of magnetism and quantum mechanics.
- Mimicking Superconductors: Cold-atom researchers are using neutral atoms in optical traps to mimic electrons in high-temperature superconductors.
- Hubbard Model: The link between solid-state and cold-atom systems is the Hubbard model, describing electrons in solids.
- Temperature Challenge: The primary hurdle is achieving low enough temperatures, as current atomic experiments are still far from cuprate phase diagrams.
- Harvard's Breakthrough: Markus Greiner's team at Harvard achieved antiferromagnetic order by cooling lithium-6 atoms in an 80-site lattice.
The Future of Quantum Gas Research
The successful cooling of quantum gases to long-range antiferromagnetic order opens up a wide range of possibilities for future research. Scientists can now use these systems to study the fundamental properties of magnetism, explore the transition between different phases of matter, and potentially discover new materials with exotic properties. The insights gained from these studies could lead to advancements in various technological fields, including quantum computing, materials science, and energy technology.