Unlocking the Secrets of Particle Production: What High-Energy Collisions Teach Us
"Delving into the heart of matter: How analyzing particle collisions at 7 TeV reveals new insights into the strong force and the building blocks of our universe."
The quest to understand the fundamental nature of matter and energy has driven physicists to conduct experiments at ever-increasing energy levels. By smashing particles together at velocities near the speed of light, scientists can recreate the conditions that existed fractions of a second after the Big Bang. These high-energy collisions provide a window into the building blocks of our universe and the forces that govern their interactions.
One of the key areas of investigation involves studying the production of different types of particles in these collisions. By carefully analyzing the types, energies, and distributions of the particles that emerge, researchers can gain insights into the strong force, which binds quarks together to form protons and neutrons, and the weak force, responsible for radioactive decay. Experiments at the Large Hadron Collider (LHC) at CERN, such as those conducted by the ALICE collaboration, have been instrumental in advancing this field.
Recent measurements have revealed collective behaviors in high-multiplicity proton-proton (pp) and proton-lead (p-Pb) collisions that resemble those observed in lead-lead (Pb-Pb) collisions. This has sparked great interest in the scientific community, prompting investigations into the origins of these intriguing phenomena. The ALICE detector, with its exceptional particle identification capabilities, is uniquely positioned to study particle production over a wide range of transverse momentum, providing critical data for understanding these complex interactions.
Dissecting Particle Production in High-Energy Collisions
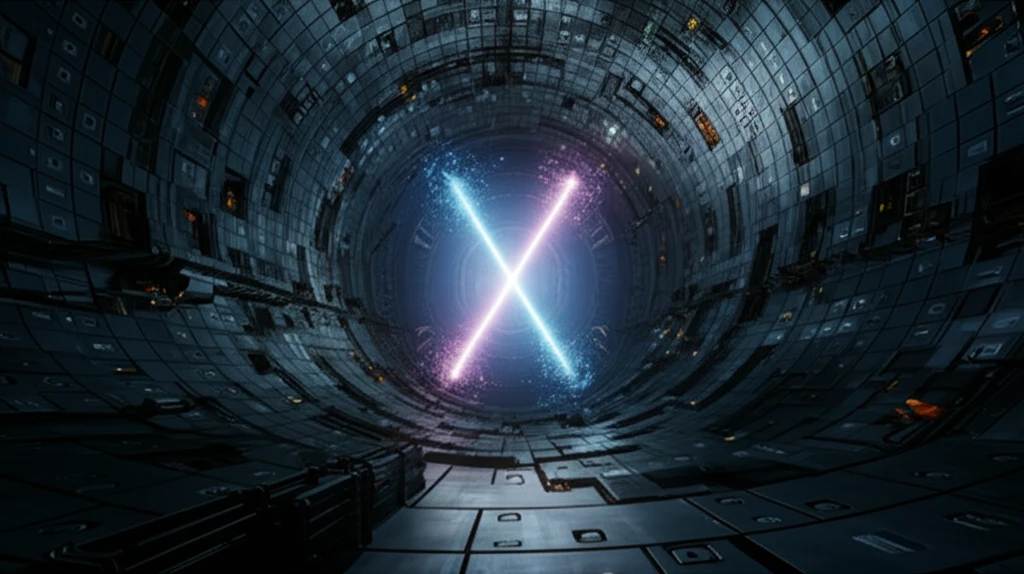
The analysis of transverse momentum spectra of various particles, including pions (π), kaons (K), protons (p), and heavier particles like Lambda (Λ), Xi (Ξ), and Omega (Ω), provides a comprehensive view of particle production dynamics. These spectra, measured as a function of event multiplicity in proton-proton collisions at a center-of-mass energy of 7 TeV, offer valuable insights into the underlying mechanisms driving particle formation. By comparing the ratios of different particle types, such as the Λ/K ratio, across different collision systems (pp, p-Pb, and Pb-Pb), scientists can identify common patterns and unique characteristics.
- Qualitative similarity in particle ratios across different collision systems.
- System size and energy density influence particle production.
- Strangeness enhancement observed in high-multiplicity events.
- Models struggle to fully explain high-multiplicity pp collision data.
The Path Forward
The study of particle production in high-energy collisions continues to be a vibrant and essential field of research. By meticulously analyzing the data from experiments like those conducted by the ALICE collaboration, scientists are steadily piecing together a more complete understanding of the fundamental forces and building blocks of our universe. Future experiments and theoretical advancements promise to further refine our knowledge and shed light on the most profound mysteries of matter and energy.