Unlocking the Secrets of Nuclear Fission: A Journey into Atomic Stability and Energy
"Delving into the heart of nuclear fission to understand how excitation energy reshapes the landscape of atomic nuclei and their decay pathways."
Nuclear fission, a process where an atomic nucleus splits into two or more smaller nuclei, has been a subject of intense study for nearly a century. This complex phenomenon involves extreme nuclear deformations, intricate nuclear structures, and the transfer of immense energy. Despite decades of research, many aspects of fission remain elusive, challenging scientists to deepen their understanding of this fundamental process.
At low excitation energies, nuclear fission uniquely combines macroscopic and microscopic aspects of nuclear matter. The shape the nucleus takes as it approaches the 'scission point'—the moment of separation—reflects a collective movement of nucleons. Simultaneously, the resulting fragment distributions are heavily influenced by the energy levels within these fragments.
While the asymmetric nature of fission distributions has been observed with a consistent mass around A~140 and atomic number Z~54 in actinides, it's only recently that the use of inverse kinematics, coupled with advanced magnetic spectrometers, has allowed for simultaneous measurements of both nuclear charge and mass distributions. This approach has opened new avenues for exploring the structure effects characterizing the scission point and relating them to the excitation energy of the fissioning system.
Deciphering the Influence of Excitation Energy
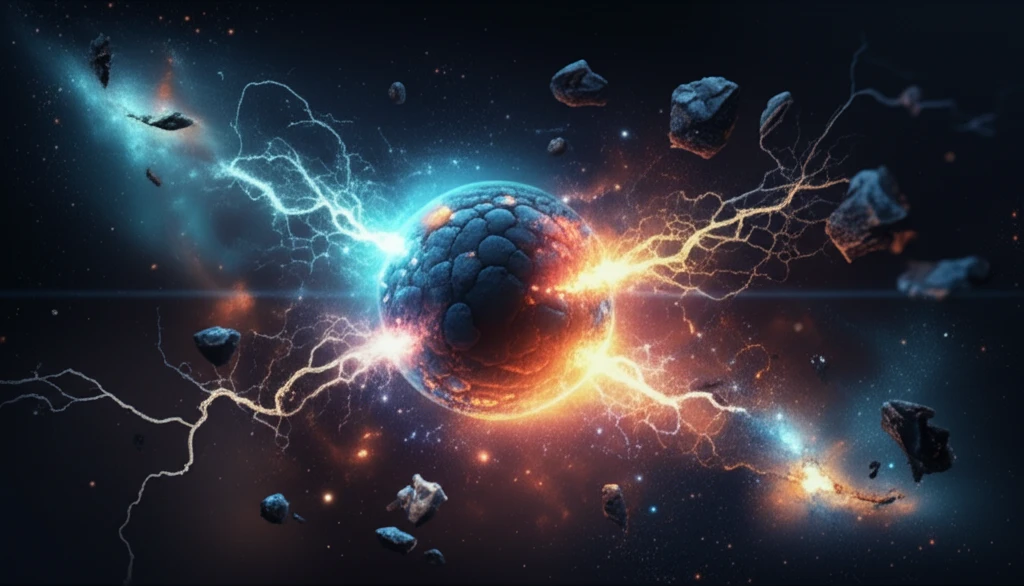
Recent experimental efforts have focused on understanding how excitation energy affects the behavior of fission fragments. By studying the fission of various actinide nuclei (such as Uranium, Neptunium, Plutonium, Curium, and Californium) at different excitation energies, scientists are beginning to unravel the underlying mechanisms that govern the process. These experiments involve bombarding a target material with a beam of heavy ions, inducing fission through transfer and fusion reactions. Sophisticated detection systems then measure the properties of the resulting fragments, including their mass, charge, and energy.
- Neutron Excess: Observing how the balance of neutrons to protons shifts with varying energy.
- Total Neutron Multiplicity: Measuring the total number of neutrons emitted during fission.
- Even-Odd Staggering: Analyzing the preference for fragments with even numbers of protons or neutrons.
The Path Forward: Refining Our Understanding
These measurements collectively highlight the profound influence of nuclear structure on the fission process and how it evolves with excitation energy. By continuing to probe the intricacies of fission, we can refine our models of nuclear behavior, enhancing our ability to predict and control nuclear reactions. This knowledge is crucial not only for fundamental science but also for practical applications such as nuclear energy and nuclear medicine.