Unlocking Fusion Energy: How Broken Symmetry Could Be the Key
"Researchers explore how asymmetries in tokamak reactors could help harness the power of energetic alpha particles"
The quest for sustainable and clean energy sources has led scientists to explore fusion power, which replicates the energy-generating process of the sun. One promising avenue is the tokamak reactor, a device designed to confine plasma—a superheated state of matter—using powerful magnetic fields. Ideally, these tokamaks should be perfectly symmetrical; however, real-world conditions introduce imperfections that researchers are now exploring as potentially beneficial.
In a perfect world, tokamak reactors would exhibit perfect toroidal symmetry, meaning they are uniform when rotated around their central axis. However, several factors can disrupt this symmetry. These include the discrete nature of the magnetic field coils, engineering inaccuracies, and the presence of magnetohydrodynamic (MHD) modes, like magnetic islands. These deviations from perfect symmetry, often called “broken symmetry,” were initially seen as a problem, but new research suggests they might hold the key to better managing energetic alpha particles within the reactor.
Energetic alpha particles, produced from fusion reactions, are crucial for sustaining the plasma temperature needed for continuous fusion. However, these particles can also escape, leading to energy loss and potential damage to the reactor walls. Therefore, understanding and controlling the behavior of alpha particles in tokamaks with broken symmetry is vital for the development of viable fusion reactors.
The Role of Broken Symmetry in Tokamak Reactors
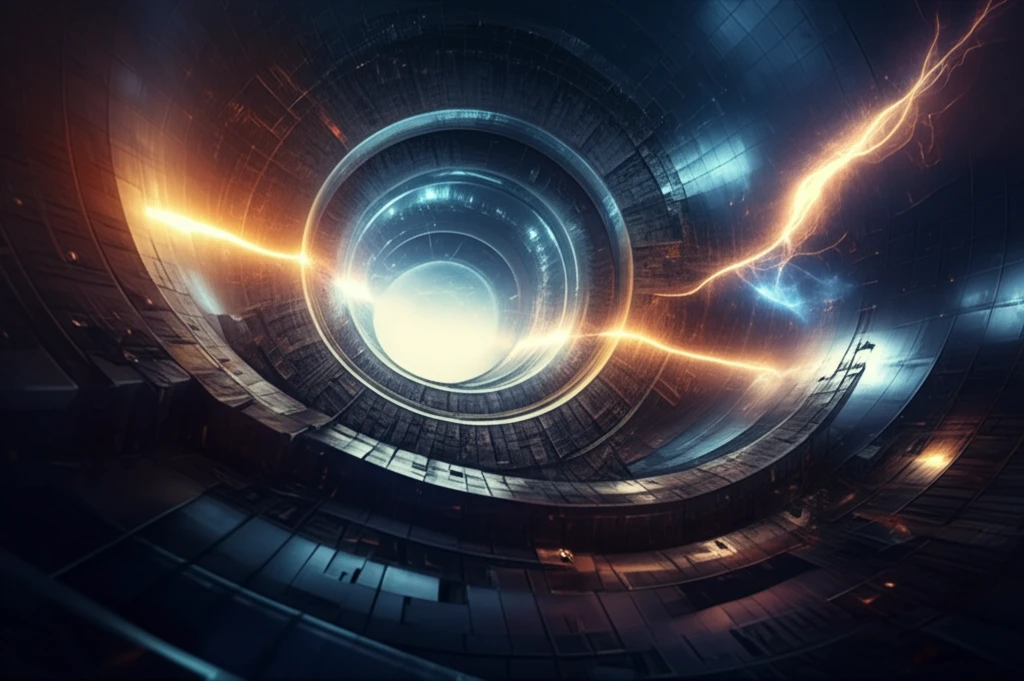
Broken symmetry in tokamaks can lead to both challenges and opportunities in controlling plasma behavior. One significant effect is the creation of drift resonances, which occur when the frequency of particle drift matches certain frequencies within the plasma. These resonances can cause particles to be lost more quickly, especially the high-energy alpha particles needed to sustain the fusion reaction. The most dangerous transport mechanism is the drift resonance caused by superbanana plateau transport fluxes.
- Enhanced Modeling: These models allow for a more accurate prediction of alpha particle behavior in fusion reactors.
- Optimization: Understanding transport fluxes helps optimize reactor designs for better energy confinement.
- Wide Applicability: The derived equations apply across a range of plasma parameters, making them versatile for different reactor conditions.
Looking Ahead
The insights gained from this research offer a pathway to refine designs and operational strategies for fusion reactors. By understanding how broken symmetry influences alpha particle confinement, scientists and engineers can work towards creating more efficient and sustainable fusion power plants. Future research will focus on refining the models for superbanana orbits and exploring advanced control techniques to harness the benefits of broken symmetry while minimizing its drawbacks. The team will separately report the transport theory for superbananas in the limit where the slowing down operator dominates.