Swarming Secrets: How Bacteria Build Spirals and What It Means for You
"Dive into the microscopic world where self-propelled bacteria chains form stunning spiral coils, revealing new insights into collective behavior and active matter."
Nature teems with collective movement. From the synchronized dance of bird flocks to the coordinated hunts of fish schools, we observe creatures moving in harmony, as if guided by a single mind. Even simpler, single-celled organisms showcase this behavior, hinting at fundamental principles governing collective motion.
Scientists have long been captivated by these phenomena, seeking to understand the rules that allow individual agents to self-organize. This pursuit spans disciplines, drawing in computational biologists, physicists, microbiologists, and engineers, each contributing unique perspectives and tools.
One particularly intriguing example is the swarming behavior of Vibrio alginolyticus, a bacterium that elongates and develops flagella when colonizing surfaces. These bacteria exhibit complex patterns, including the formation of striking spiral coils. Recent research delves into the dynamics of these self-propelled chains, exploring how bending elasticity and density influence the creation of these fascinating structures.
The Building Blocks of Bacterial Spirals
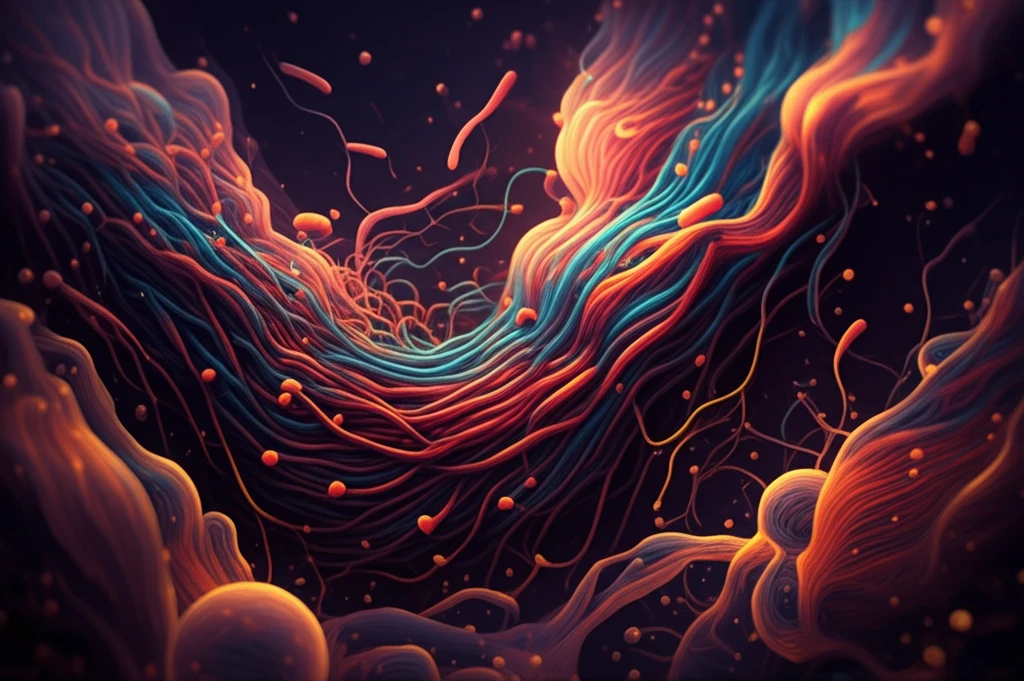
The recent study employs Brownian dynamics simulations to model self-propelled chains, mimicking the behavior of V. alginolyticus. These simulations factor in excluded volume interaction—the physical space each chain occupies—and, crucially, the bending elasticity of the chains. By adjusting the chains’ flexibility, researchers can observe how these properties influence cluster formation and the emergence of spiral coils.
- Hydrodynamic flows are negligible.
- Active propulsion is modeled as a constant force acting on the cell body.
- The only interaction between chains is excluded volume.
Unraveling the Mysteries of Swarm Dynamics
The study highlights the intricate relationship between individual bacterial properties and collective behavior. By understanding how bending elasticity and density influence spiral formation, scientists can gain a deeper appreciation for the principles governing living systems. This knowledge holds promise for diverse applications, from designing novel bio-inspired materials to engineering microbial communities for specific tasks.