Shine Brighter: How Proton Implantation is Revolutionizing Glass Waveguides
"Unlock the Potential of Near-Infrared Light with Advanced Glass Waveguide Technology."
In the fast-evolving world of photonics, the ability to manipulate light at a microscopic level is paramount. Optical waveguides, which act as tiny conduits for light, are essential components in various technologies, from high-speed internet to advanced sensors. Recent advancements in materials science have focused on enhancing the performance and stability of these waveguides, particularly those made from silicate glass.
Silicate glasses are popular because of their high solubility for rare-earth ions like Erbium (Er3+) and Ytterbium (Yb3+), which are essential for creating amplifiers and lasers. When these ions are integrated into glass waveguides, they can boost signal strength and enable efficient light emission. However, the challenge lies in creating waveguides that are not only efficient but also thermally stable, meaning they can maintain their properties under varying temperatures.
A groundbreaking technique known as proton implantation is emerging as a powerful solution to this challenge. By bombarding silicate glass with protons, scientists can alter the refractive index of the material, creating well-defined pathways for light. This method not only improves the optical properties of the glass but also enhances its thermal stability, making it suitable for demanding applications. Let's explore how this technology is transforming the landscape of optical devices.
The Science Behind Proton Implantation
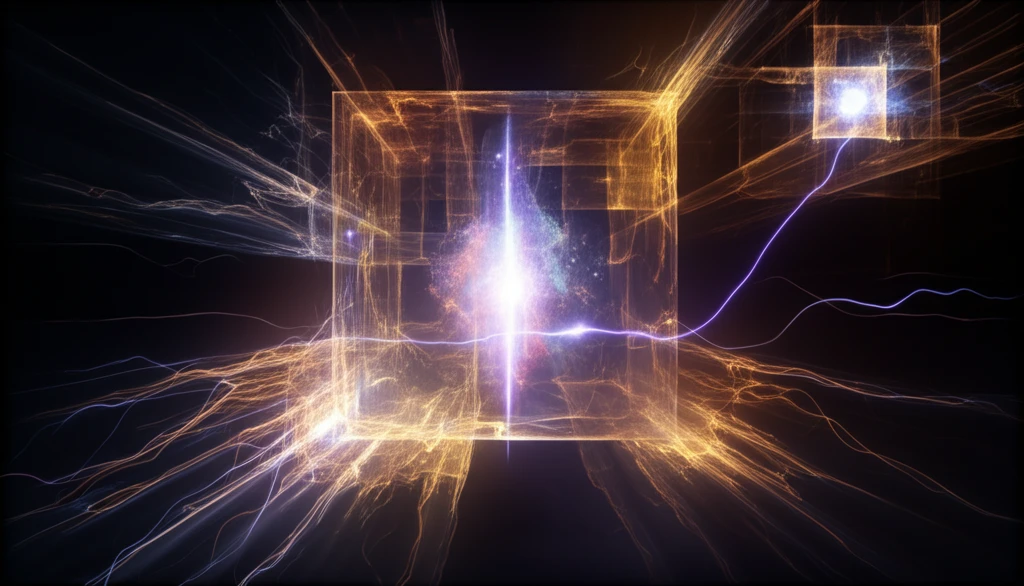
Proton implantation involves firing high-energy protons into a material to modify its structure and properties. When protons collide with the silicate glass, they create a region of higher refractive index, which acts as a core for the waveguide. This core guides light by confining it within this region, similar to how fiber optic cables transmit light over long distances. The precision of this technique allows for the creation of waveguides with specific dimensions and characteristics, optimized for different wavelengths of light.
- Precise control over waveguide dimensions and refractive index.
- Enhanced thermal stability, ensuring consistent performance.
- Improved optical properties for efficient light transmission.
- Compatibility with rare-earth ions for amplification and lasing applications.
The Future of Glass Waveguides
Proton implantation is a promising method for creating high-performance glass waveguides with enhanced optical properties and thermal stability. As technology advances, these waveguides are expected to play an increasingly significant role in telecommunications, integrated photonics, and other applications that rely on the precise manipulation of light. Further research and development in this field could unlock new possibilities for optical devices, making them smaller, more efficient, and more reliable.