Nuclear Fusion's Future: How Time-Dependent DFT Could Revolutionize Energy
"Unlocking fusion energy: A closer look at time-dependent density-constrained DFT and its potential to reshape our understanding of nuclear interactions"
The pursuit of nuclear fusion as a viable energy source has driven decades of research, aiming to replicate the reactions that power the sun. Understanding the interactions between atomic nuclei is fundamental to achieving controlled fusion, opening doors to abundant, clean energy. Recent advancements in computational nuclear physics offer fresh perspectives on these complex processes.
One promising avenue is the use of time-dependent density-constrained Density Functional Theory (DFT). This method allows scientists to model the behavior of nuclei during fusion reactions with unprecedented accuracy. By simulating these interactions, researchers gain crucial insights into the forces at play and the conditions necessary for successful fusion.
This article explores how time-dependent density-constrained DFT is transforming our understanding of nuclear fusion, providing a detailed look at the method, its applications, and its potential to overcome existing challenges in the field.
Decoding Time-Dependent Density-Constrained DFT
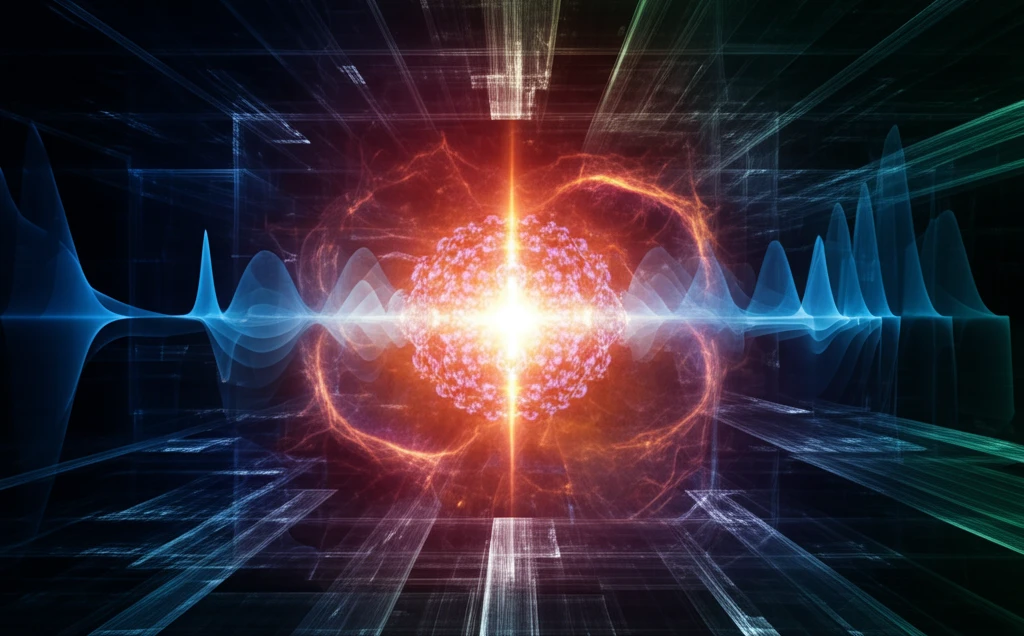
Time-dependent density-constrained DFT is a sophisticated computational technique used to simulate the dynamics of nuclear reactions. Traditional methods often simplify the interactions between nuclei, but DC-TDHF offers a more nuanced approach. By incorporating time-dependent calculations, it captures the evolving nature of nuclear densities during fusion, giving a more realistic picture of the process.
- No need for constraining operators, allowing the system to evolve naturally.
- Inclusion of effects like neck formation, mass exchange, and internal excitations.
- Accounting for deformation effects and nuclear alignment in deformed systems.
- Comprehensive calculation of fusion barriers in the mean-field limit.
The Future of Fusion
Time-dependent density-constrained DFT is a crucial tool in the ongoing quest for sustainable fusion energy. As computational power increases and theoretical models become more refined, this approach promises even greater accuracy and predictive capability. By continuing to explore and refine these methods, scientists are steadily moving closer to unlocking the full potential of nuclear fusion, potentially revolutionizing the world's energy landscape.