Decoding Ice: How Scientists Are Modeling Its Strength Under Pressure
"New research unveils a comprehensive model for understanding ice behavior under extreme conditions, crucial for Arctic exploration and structural safety."
The Arctic, once a remote frontier, is now a focal point for global exploration and resource extraction. As sea routes open and offshore ventures expand, understanding the fundamental properties of ice becomes paramount. The interaction between ice and ships or marine structures poses significant engineering challenges, necessitating accurate predictive models of ice behavior.
For decades, scientists have grappled with the complexities of ice, a material whose strength and deformation characteristics are notoriously difficult to predict. Unlike steel or concrete, ice responds dramatically to changes in temperature, pressure, and the rate at which it is stressed. This sensitivity demands sophisticated models that account for these variables to ensure the safety and reliability of Arctic operations.
Recent research published in Ships and Offshore Structures introduces a novel ice material model designed to do just that. This model aims to capture the intricate interplay of strain rate, temperature, and confining pressure, offering a more realistic representation of ice behavior under the extreme conditions encountered in the Arctic and other cold regions.
What Factors Influence Ice Strength? Unveiling the Model's Components
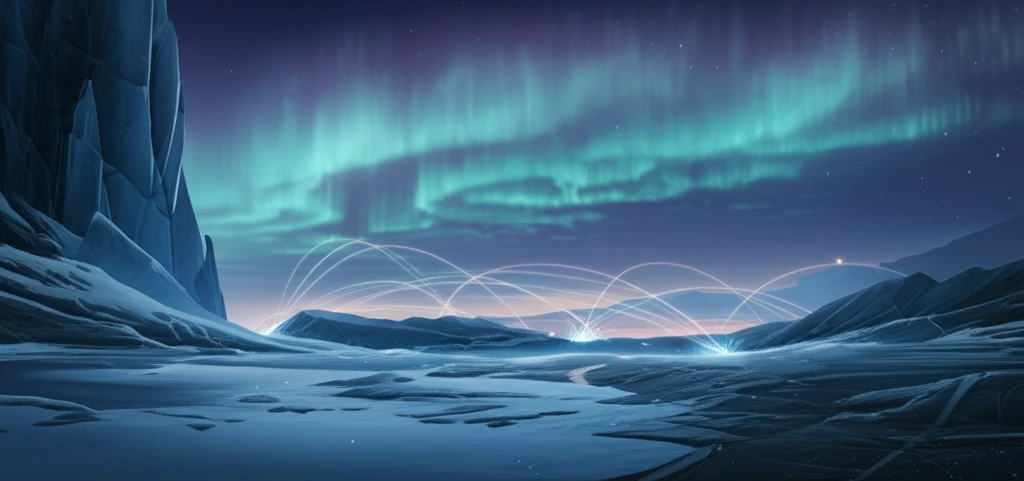
The newly developed ice material model is built upon the fundamental principle that ice deformation can be categorized into recoverable and unrecoverable components. The recoverable portion is represented by elastic and delayed elastic models, while the unrecoverable deformation is modeled with a viscous model. This viscous model links stress to strain rate, temperature, and confining pressure, capturing how these factors influence ice's resistance to deformation.
- Isotropy: Assumes the ice material has uniform properties in all directions.
- Deviatoric Behavior: Models shape changes using viscous, elastic, and delayed elastic laws.
- Temperature Influence: Incorporates temperature effects on viscous and delayed elastic behaviors.
- Volumetric Behavior: Represents volume changes using the elastic model, accounting for how hydrostatic stress affects deviatoric behavior.
Implications for Arctic Engineering and Beyond
This improved understanding of ice mechanics is crucial for a wide range of applications, from designing safer ships and offshore platforms to predicting the stability of ice sheets and glaciers. As the Arctic continues to open up to development, models like this will play an increasingly important role in ensuring the safety and sustainability of human activities in this challenging environment.