Decoding Cell Signals: How Understanding Cellular Conductivity Could Revolutionize Health
"Scientists develop a quantitative model to unlock the secrets of cell communication and its implications for diseases like cancer and diabetes."
Our bodies are composed of trillions of cells, each acting like a miniature city, constantly communicating and working together to keep us alive and functioning. A key aspect of this cellular communication involves the movement of ions—electrically charged particles—within and between cells. This movement, known as ion transport, influences a cell's cytoplasm conductivity, acting as a barometer of its overall health.
Changes in cytoplasm conductivity can indicate various physiological shifts, including the progression of diseases like cancer and the onset of apoptosis (programmed cell death). Scientists have long sought to understand and model these changes to gain insights into disease mechanisms and potential treatments. Now, researchers are making strides in developing sophisticated models that predict how alterations in ion transport affect cellular conductivity, potentially revolutionizing how we approach disease diagnosis and treatment.
One such breakthrough comes from a team of scientists who have developed a quantitative model focusing on Chinese Hamster Ovary (CHO) cells. These cells, commonly used in biopharmaceutical research, serve as an excellent model for understanding fundamental cell behaviors. By studying CHO cells, researchers can develop models applicable to a wide range of mammalian cells, providing insights into various health conditions.
The Science Behind Cellular Conductivity
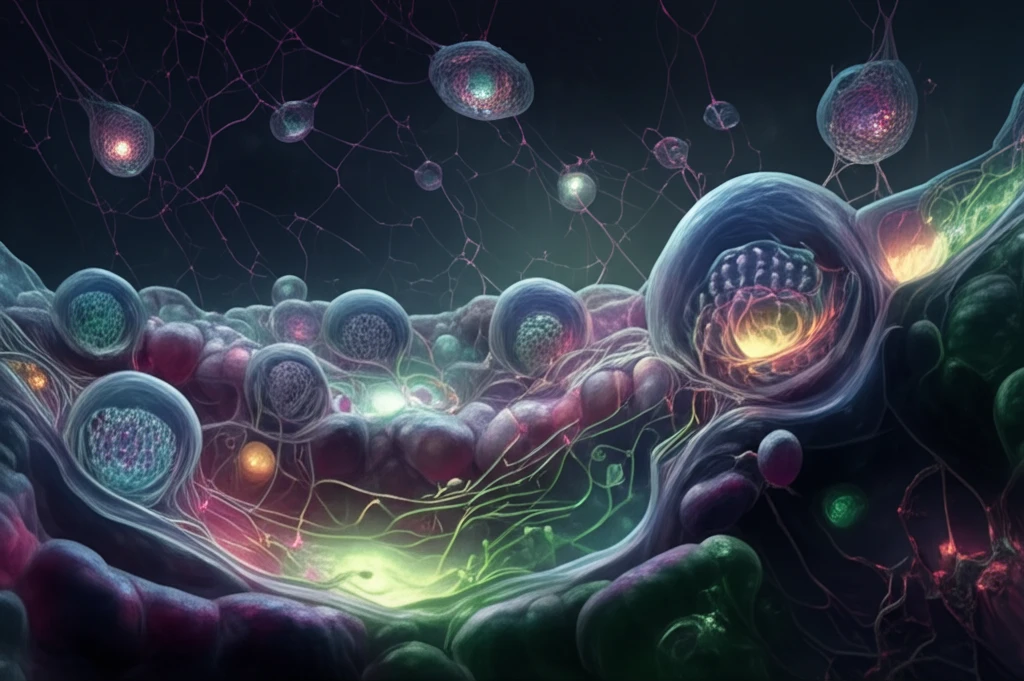
To build their model, the scientists focused on key components that influence ion transport: Na+/K+ ATPase pumps and ion channels. These act as gatekeepers, controlling the flow of ions across the cell membrane. Using a technique called flux assay, the team measured the movement of ions in CHO cells to determine cell-specific parameters, essentially creating a detailed profile of how these cells manage ion transport.
- CHO cells can provide critical insights for understanding mammalian cells.
- Ion concentration changes can indicate impaired cellular functions, such as apoptosis.
- Ion transport models can predict temporal changes in cytoplasm conductivity.
- Measuring electrical properties can help assess biological and physiological changes.
Implications and Future Directions
This quantitative model opens new avenues for understanding the effects of various processes, such as apoptosis or changes in external media ion concentration, on the cytoplasm conductivity of mammalian cells. In the future, the team is planning to create algorithms that could predict the onset and progression of the diseases and the outcome of treatments.