Crystal Clear: How Scientists Are Tuning Semiconductor Performance with Nickel
"Unlocking new potential in semiconductor technology through innovative crystal transformation techniques in nickel complexes."
Imagine a world where our electronic devices are not only more powerful but also more adaptable, thanks to advancements at the molecular level. Polymorphism, the ability of a solid material to exist in multiple crystal forms, is a phenomenon that has long intrigued scientists. These different forms can exhibit a range of properties, from color and solubility to magnetic and electrical behaviors. Recent studies have focused on how to harness this variability, particularly in metal-organic hybrid crystals, to revolutionize semiconductor technology.
Semiconductors are the backbone of modern electronics, found in everything from smartphones to solar panels. Traditionally, research has centered on organic oligomers and polymers. However, the spotlight is now turning to coordination complexes—structures where a central metal atom is surrounded by ligands (molecules or ions)—offering new avenues for creating superior semiconductor materials. These complexes, especially those with planar, conjugated ligands, have shown promise in enhancing the performance of organic field-effect transistors (OFETs), light-emitting diodes (LEDs), and photovoltaic devices (PVs).
One particularly promising area involves Schiff bases, conjugated compounds known for their interesting optoelectronic properties. When used as semiconductor materials and combined with the concept of crystal transformation, these bases could lead to significant advancements. The key is understanding how altering the crystal structure of these materials can fine-tune their semiconducting properties, opening up possibilities for new and improved modification methods.
Tuning Nickel Complexes Through Crystal Transformation
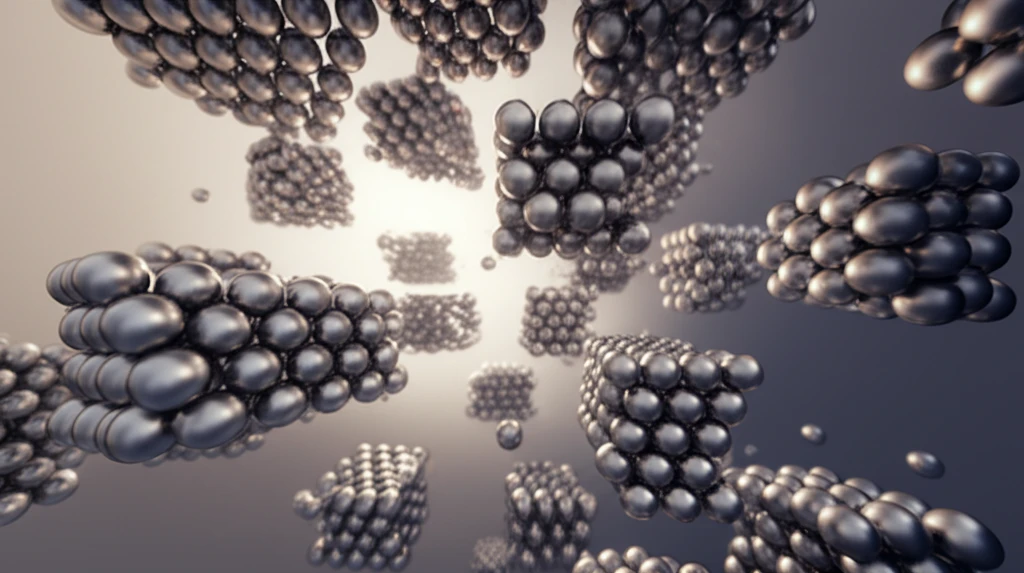
A groundbreaking study has explored the crystal transformation between two polymorphs of a square-planar nickel complex, known as NiL2. The two forms are a green crystal (1-G) and a red crystal (1-R). NiL2 (L = 2-ethoxy-6-(N-methyliminomethyl)phenolate) and its tuning effect on semiconductor properties were meticulously examined both experimentally and theoretically. The transformation occurs when 1-G is heated to 413 K (approximately 140°C), converting it to 1-R. Conversely, soaking 1-R in various solvents reverts it back to the 1-G form. This reversible transformation offers a unique way to manipulate the material's properties.
- Heating 1-G: Converts it to 1-R at 413K.
- Soaking 1-R: Reverts it back to 1-G in solvents.
- Conductivity Increase: Significant increase upon heating 1-G.
- Crystal Changes: Dramatic changes in crystal dimensions and packing models.
The Future of Semiconductor Tuning
This research not only enhances our understanding of crystal transformations but also paves the way for designing new semiconductor materials with superior performance. By manipulating the crystal structure of nickel complexes, scientists can achieve precise control over their electrical properties, opening up exciting possibilities for more efficient and versatile electronic devices. This approach offers a promising avenue for future innovations in semiconductor technology, potentially leading to breakthroughs in various applications.